subscribe to our mailing list:
|
SECTIONS
|
 |
 |
 |
Evolving Immunity
A Response to Chapter 6 of Darwin's Black Box
By Matt Inlay
created May 31, 2002, Last Modified: June 21, 2002, posted June 30, 2002
Introduction
Immune systems are generally characterized by their
ability to distinguish between self and non-self cells,
tissues, or molecules, and to eliminate the non-self
(Janeway 2001). The mammalian immune system is an
incredibly complex and intricate system that can recognize
non-self and provide protection from a wide variety of
pathogens. While there is a high degree of
interconnectivity between its components, the immune system
can be loosely divided into two subsystems, the innate and
the adaptive immune systems. In general, innate immunity
is a non-specific, inducible response to pathogens. It is
immediate in action, yet short-lived. On the other hand,
the adaptive immune system is much more specific, but takes
longer to activate. It also features immunological memory,
and can augment itself to respond more quickly and with
greater specificity to future infections of similar
pathogens. Both systems work together to provide
protection against a diverse and rapidly-evolving array of
pathogens.
While much is known about the evolution of the
immune system, the details of its origin remain to be
elucidated. The innate immune system is the more ancient
of the two systems, with roots deep in the deuterostome
branch of the bilaterians, roughly one billion years ago.
Conversely, the adaptive immune system appeared more
recently and quite suddenly, around 450 million years ago
with the emergence of the gnathostomes, more commonly
called the jawed vertebrates. Because of its high degree
of complexity and interconnectivity, the mammalian immune
system has been labeled as "irreducibly complex", and its
evolution and origin through "Darwinian" mechanisms
challenged[i] .
Michael Behe, in his book Darwin's Black Box
(Behe 1996 ), defines irreducible complexity (IC) as:
"[A] single system composed of several well-matched,
interacting parts that contribute to the basic function,
wherein the removal of any one of the parts causes the
system to effectively cease function." (page 39).
Unfortunately, each of the terms "system",
"well-matched", "interacting", "parts", and "function" can
have multiple meanings, depending on the context, so
nonarbitrarily labeling a system as IC is not an easy
task. For instance, "system" in Behe's definition differs
significantly from the same word in the context of "immune
system". His use of "system" refers specifically
to biochemical systems. What is the difference? The components,
or parts of a biochemical system are molecular in
nature, like proteins or complexes of molecules. "System" as is
used in immune system refers not just to molecules, but
to the cells and tissues involved in conferring
immunity. The immune system as a whole contains
numerous biochemical systems within it. It is important to
note this distinction because Behe only addresses
biochemical systems as IC.
Systems, whether biochemical or not, are often defined
according to their function. However, the term "function"
is also nebulous. The "function" of the immune system is
rather broad, encompassing any action involved in either
immune recognition or response. Even the skin is
considered to be part of the immune system. The "function"
of a biochemical system is much more specific. Here,
function refers to a specific biochemical function, such as
the formation of a blood-clot, the spinning of a flagella
to produce locomotion, or the synthesis of a biomolecule
from a precursor. This type of function usually consists
of a series of biochemical reactions. This is important
because Behe is trying to make a specific argument about
the origin and evolution of IC biochemical systems.
Immediately after defining IC, Behe states:
"An irreducibly complex system cannot be produced
directly (that is, by continuously improving the initial
function, which continues to work by the same mechanism) by
slight, successive modifications of a precursor system,
because any precursor to an irreducibly complex system that
is missing a part is by definition nonfunctional. An
irreducibly complex biological system, if there is such a
thing, would be a powerful challenge to Darwinian
evolution. Since natural selection can only choose systems
that are already working, then if a biological system
cannot be produced gradually it would have to arise as an
integrated unit, in one fell swoop, for natural selection
to have anything to act on."
Behe is trying to suggest that because of the nature of
IC, biochemical systems that possess this quality could not
have evolved through random mutation and natural selection,
or "blind-watchmaking". Essentially, Behe is using IC to
disprove evolution. As an alternative, Behe suggests that
the only way that IC biochemical systems could have
originated is through intelligent intervention. He offers
as evidence the fact that all IC systems whose origins are
known were designed by intelligent agents, us.
There are many problems with Behe's argument.
Basically, Behe is saying that because we do not know how
an IC system could have evolved, it didn't evolve. This
type of argumentation is referred to as an argument from
ignorance. The problem with arguments from
ignorance is that they make a conclusion based on a lack of knowledge. There is still much we do not
know about evolution. What seems like a conundrum right now
may be easily resolved in the future. Biology, and science
in general, is filled with mysteries and unknowns. The
whole point of science is to seek answers to
those questions. Secondly, he tries to use arguments against evolution
as evidence for intelligent design. Negative arguments against one
theory do not necessarily provide evidence
for a particular alternative. It would be like trying
to show how safe a Honda Civic is by providing
evidence that a Toyota Corolla is unsafe. No matter how unsafe
the Corolla is, it says nothing about the Civic. Negative
argumentation is scientifically useless without an alternative that
can be positively tested. These fallacies
do not rule out the possibility that Behe
has raised an interesting and useful challenge to our current
understanding of evolution. However, a closer look
reveals he hasn't.
In chapter 6 of Darwin's Black Box, entitled "A Dangerous World", Behe outlines three IC biochemical
systems in the immune system; clonal selection, V(D)J recombination, and the
complement cascade. However, in his analysis of each of these systems,
Behe makes critical mistakes. For the process of clonal selection, Behe
targets the antibody molecules, which have the ability to rearrange their genes,
and to switch from a membrane-bound form to a secreted form. Behe assumes
that an antibody molecule missing one of these features would be useless.
However, there are many examples of proteins that do not undergo rearrangement,
but are able to switch forms. Additionally, there is a gene closely
related to antibodies which can also undergo rearrangement, but does not switch
forms. Behe neglects to mention either of these intermediate-like
molecules. Instead, he scrutinizes the process of antibody gene
rearrangement as the second IC system. He describes the numerous features
of this system and claims there is no pathway through which it
could have evolved. However, he assumes that the only selectable
function for the genes involved in rearrangement is rearrangement.
There are well-studied systems that bear remarkable similarity to
V(D)J recombination that have nothing to do with immunity. He
fails to recognize the existence of these other systems,
nor realizes that most of the
components of recombination are already present there. Finally, in
his description of the third IC system, Behe mistakenly assigns
a single function to the complement pathway. He fails to realize
that the complement system has several different functions, and several
mechanisms of activation. This would allow for a
gradual, stepwise accumulation of parts and
functions. In fact, many other groups of organisms
possess a complement system that lacks his primary function. According
to Behe's definition of irreducible complexity, those
systems shouldn't exist.
In each case, Behe claims that a lack of articles in the
scientific literature provides evidence that these systems
could not have evolved. However, there are hundreds to
thousands of articles published on these systems. Much was
known about the evolution of each of these systems at the
time Darwin's Black Box was published.
Additionally, much has been learned since then. This
article will attempt to summarize the existing knowledge
about the origins of these systems, and propose a possible
pathway for their evolution through a Darwinian mechanism.
It is not necessary to "prove" that these systems evolved.
Because the essence of his argument is "how could
this have evolved?", this article merely seeks to provide
an answer to that question. One issue that will not be
discussed is whether or not these systems are actually
irreducibly complex. The term is not very useful to the
discussion of origins because of the ambiguities
surrounding its definition. In fact, IC as a term has
failed to make much of an impression on the scientific
community, as a search on the publication database PubMed,
fails to turn up any references to "irreducible
complexity"[ii] .
IC system I: Clonal selection
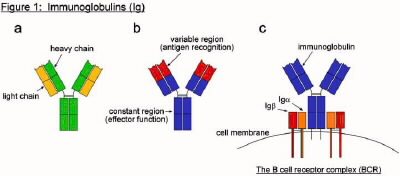
The B cell receptor complex (BCR)
The first system Behe describes in chapter 6 is the
process of clonal selection, which is how the adaptive immune system detects
non-self molecules and initiates a response. This system focuses on a
protein called immunoglobulin (Ig), and the complex it is part of, called the B
cell receptor complex (BCR). Immunoglobulins, also called antibodies, are
Y-shaped proteins that can recognize and bind foreign molecules (Figure
1a,b). They are produced by lymphocytes called B cells, and are initially
expressed on the cell surface. In its membrane-bound form, an
immunoglobulin molecule binds to two additional proteins, Iga and Igb, to form the BCR (Figure 1c). The target of an
immunoglobulin is referred to as its antigen (Ag). Antigen can be
essentially any molecule, though there is a preference for proteins.
Because of the way in which immunoglobulins are produced, the specific antigen
that each Ig recognizes is different in each B cell. This occurs through a
process of gene rearrangement called V(D)J recombination, which will be
explained later. When an unactivated, or naïve, B cell's Ig recognizes its
particular antigen, it sends a signal back into the interior of the cell to
initiate activation. This signal is transmitted via a series of
modifications through a series of "messenger" proteins, which ultimately
trigger the transcription of a panel of genes involved in B cell
activation. In order to become fully activated, further interaction with a
second class of lymphocyte, called T cells, is required. Once the B cell
is activated it proliferates and differentiates into a plasma cell, and the
function of the immunoglobulin changes from recognition to response. The
immunoglobulin switches from a membrane-bound form (mIg) to a secreted form
(sIg), where it can diffuse throughout the tissues of the host
organism and there bind its cognate antigen. The only difference between
the two forms is at the very tip of the bottom of the Y shape, which corresponds
to the very tail end of the gene. The switch from mIg to sIg production
results from a switch between two alternative RNA splicing patterns, such
that the terminal coding exon directs either the translation of a
membrane-bound or soluble C-terminal domain, respectively (Figure 2).
The process of amplifying a particular B cell (or subset of B cells) from
amongst billions based on its antigen specificity is called clonal selection
(Figure 3). This process allows the immune system to choose from which B
cell (or subset of B cells) it will mount an immune response. Additional
discussion of the clonal selection system in the context of irreducible
complexity can be found in the web article: The Evolution
of Improved Fitness

Behe summarizes this first system as a group of 3
components (page 125):
membrane-bound form of the antibody
messenger
secreted form of the antibody
Behe has this to say about the evolution of the clonal
selection system:
"A cell hopefully trying to evolve such a system in
gradual Darwinian steps would be in a quandary. What should it do
first? Secreting a little bit of antibody into the great outdoors is a
waste of resources if there's no way to tell if it's doing any good.
Ditto for making a membrane-bound antibody. And why make a messenger
protein first if there is nobody to give it a message, and nobody to receive
the message if it did get one? We are led inexorably to the conclusion
that even this greatly simplified clonal selection could not have come about
in gradual steps." (Page 125)

Switch forms or rearrange?
So the question is, could the clonal selection
system have evolved? Certainly. One thing to realize is that the
immunoglobulins are not the only molecules that can undergo gene rearrangement
to produce a diverse set of antigen-receptors. T cell receptors (TCRs) are
antigen-receptors quite similar to immunoglobulins. However,
TCRs exist only in a membrane-bound form, yet are able to
carry out their particular function without the need to leave the cell
surface. Their receptors are used only for the detection of foreign
antigens, and do not directly mediate an effector response. So even if
immunoglobulins would be unable to effectively carry out their job if they were
restricted to the membrane-bound form, they could have evolved from a TCR-like
gene, which does not require secretion. If that occurred, then the ability
to rearrange and the ability to switch forms could evolve in separate steps,
with each step offering a selectable advantage.
It remains unclear whether a TCR-like gene appeared first
or an Ig-like gene. However, it is also possible that the ability to
rearrange came after the evolution of the alternative
splicing pattern, resulting in the ability to switch. This would require
that antigen receptors with a single specificity be effective mediators of
immunity. Could this have occurred? Absolutely. There are several
families of innate receptors that do not rearrange, called pattern recognition
receptors (PRRs) (Medzhitov and Janeway 1997). Bacteria
possess many unique and essential surface molecules that are distinct from
metazoan surface molecules. For example, organisms ranging from insects to
humans bear numerous receptors capable of recognizing the bacterial cell wall
component lipopolysaccharide (LPS) (Fenton and Golenbock 1998). Examples
include the macrophage scavenger receptor, CD14, and the ß2-integrins.
Binding to these receptors induces a variety of responses, from the release of
inflammatory agents to the phagocytosis of the LPS-carrying particle. Some
of these receptors are membrane-bound and some are secreted.
Interestingly, there are some that can exist in both forms, such as CD14.
So here we have examples of receptors that do not rearrange, can be
membrane-bound, secreted, or both, and can mount an effective immune
response.
Recently, a gene was discovered in the silkmoth Hyalophora cecropia whose expression is upregulated upon
exposure to LPS (Sun, Lindstrom et al. 1990). The
gene product, a protein called hemolin, is secreted into the hemolymph
(the invertebrate "blood") and can bind to the most toxic region of LPS (Daffre and
Faye 1997). This protein is also constitutively expressed in a
membrane-bound form on the surface of hemocytes (the invertebrate white blood
cell equivalents). Interestingly, the structure of this protein was found
to contain 4 Ig domains, and is a member of the Ig superfamily (Lindstrom-Dinnetz, Sun et al.
1995). Ig domains are a type of 3D protein structure first identified
in immunoglobulins, consisting of 7 or 8 ß-pleated sheets. Additionally, the crystal
structure was recently solved and revealed a shape loosely similar
to an immunoglobulin arm (Su, Gastinel et al. 1998). However, sequence
analysis shows that hemolin most likely does not represent a precursor to
immunoglobulins as the type of Ig-domain it possesses (the I-set) is one
not found in vertebrates. Hemolin does show similarities both structurally
and functionally to cell-adhesion molecules, and also contains some
cell-adhesion activity. This dual function, both for immune response and
cell-adhesion, suggests that immune molecules arose from cell-adhesion molecule
precursors. Hemolin is a clear example of an inducible immune molecule
that can exist in both membrane-bound and secreted forms.
Immunoglobulin signal transduction
When an antibody recognizes an antigen, it sends a signal
back into the cell, which "primes" the cell for activation. But what is
the nature of this signal? How exactly does the binding of antigen, which
occurs on the exterior of the cell, induce a signaling cascade on the
interior? The answer lies in the branched nature of the
immunoglobulin. As previously stated, immunoglobulins are loosely shaped
like a Y, with two arms sticking out into the extracellular space, and the
bottom embedded into the membrane. Associated with each Ig molecule are
the two accessory proteins Igα and
Igß. These accessory
molecules have long cytoplasmic tails, and contain motifs specialized for
attracting the type of kinases required to initiate the cascade. These
motifs are called ITAMs (Immunoreceptor Tyrosine-based
Activation Motifs). These motifs serve as targets for
protein tyrosine kinases, which phosphorylate certain tyrosine residues on
the ITAMs. Because most antigens have multiple epitopes (i.e. multiple
binding sites for antibodies), and because the immunoglobulins have multiple
arms, the recognition of an antigen results in a clustering of
immunoglobulins in the area of the membrane where the antigen comes in
contact with the B cell (Figure 4). This process is often
called crosslinking, and is the key to activating the cell. Visually,
it resembles a "hands across America", where each arm of the Ig is linked
to an arm of another Ig, and so on and so on. As more and more Igs cluster
around the antigen, a family of kinases, called the src family kinases, which
are loosely associated with the cytoplasmic tails of Igα and Igß, begin to phosphorylate the ITAMs. Because
the src kinases bind to phosphorylated tyrosines, this sets up a positive
feedback loop, and more and more src kinases are recruited to the site.
The phosphorylated tyrosines also bind another kinase called syk. The
newly bound syk kinase begins to phosphorylate and activate other syk
molecules, which in turn phosphorylate and activate the next molecule
in the signaling cascade, thereby propagating and amplifying the signal.
The downstream components of this cascade are also expressed in other cell
types, and are considered more general signal transducers. In this
way, a general transduction pathway can be co-opted by more specific
signal molecules. This leads to the activation of transcription
factors (TFs), which then enter the nucleus and initiate the transcription of
genes involved in cell proliferation and differentiation. In this
particular situation, the B cell becomes "primed" for activation by T cells.
Most of the "messengers", or components of the BCR
signaling pathway are homologous to many other signaling pathways present in
other tissues and in other organisms. For instance, one of the final
components in the pathway is the activation of the transcription factor NF-κB. This transcription factor is
used in multiple tissues in a wide variety of organisms to transcribe numerous
immune-related genes. The DNA sequence recognized by NF-κB is GGGRNNYYCC (where R = purine, Y =
pyrimidine, and N = any base). Interestingly, NF-κB is also a key TF in the insect immune system, and is
activated by the toll receptor pathway leading to transcriptional activation of
many insect immune-related genes. Even in mammals many innate receptors,
including the LPS receptor CD14, activates transcription through NF-κB. This also proceeds through a
toll-like receptor. For an antigen-receptor to co-opt a pathway such as
this, it would only need to link its antigen-receptor to the start of that
network. This could be achieved by acquiring accessory molecules with
ITAMs. However, until recently it was thought that ITAMs only existed in
accessory molecules to Igs and TCRs. But recently, a protein that contains
two ITAMs was discovered in the invertebrate ascidians (tunicates) (Takahashi, Ishikawa et al. 1997). This
protein is required for cellular defense mechanisms, such as phagocytosis, and
is mediated by hemocytes. Additionally, more recent experiments
demonstrated that the activity of this protein is dependent upon tyrosine
phosphorylation (Ishikawa, Azumi et al. 2000). While the
tyrosine kinase responsible for phosphorylating the ITAMs of this protein
remains to be discovered, it strongly suggests that a precursor system, with
ITAM-bearing accessory molecules, tyrosine kinases, and NF-κB, was already in place long before the
immunoglobulins appeared.
Conclusion for system
I
Using the information presented, a scenario for the
evolution of the immunoglobulin activation/secretion pathway can be
postulated. (1)It begins first with an innate membrane-bound receptor that
can induce the transcription and release of antimicrobial peptides upon
interaction with a specific foreign antigen (such as LPS). (2)A mutation
results in an alternative mRNA splice variant that directs the synthesis of a
secreted form, providing a selectable advantage. (3)Finally, the ability
to generate a diverse repertoire of antigen-receptors through gene rearrangement
evolves. This scenario is fully consistent with the known literature, and
the provided examples demonstrate the feasibility and selectability of each
step: (1)The LPS receptor in drosophila (or mammals), (2)hemolin in moths,
and (3)immunoglobulins. This model is rich with research opportunities to
either confirm or refute it, a feature distinctly lacking in Behe's model.
IC system II: The Rearranging Antigen Receptor
Loci
There are on the order of one billion B cells circulating
in a human being at any given time. Each of these B cells expresses an
immunoglobulin with a different specifity (actually, the surface of the B cell
has several thousand of its distinctive Ig attached to it). How is this
diversity achieved? The genes encoding immunoglobulins (and TCRs) are
composed of multiple gene segments, which are cut-and-pasted together in each B
cell to form a functional Ig gene through a process called V(D)J recombination,
or gene rearrangement (Figure 5). In non-lymphocytes, which do not undergo
rearrangement, the gene segments are clustered in 2 or 3 groups. For
example, at the Ig heavy chain locus, there is a cluster of about 50 V gene
segments, a second cluster of 27 D gene segments, and third cluster of 6 J
segments. During B cell development, a random gene segment
from each cluster is joined to form a continuous V-D-J gene. This
forms the antigen-binding region of the heavy chain. From this process
alone, 8100 different combinations of heavy chains can be produced. In
addition, the joining process is imprecise, and several nucleotides can be added
or removed at each junction. These junction points actually form a portion
of the antigen-binding pocket at the tip of the immunoglobulin.

In the last 12 years, a great deal has been learned about
the mechanism of V(D)J recombination. It essentially involves two
proteins, called RAG-1 and RAG-2. RAG stands for Recombination
Activating Gene. These two proteins together recognize
special sequences flanking each gene segment, called recombination signal
sequences (RSS). The RAG proteins bind to the RSSs of two gene segments,
then bring them in close proximity to each other and cleave the DNA precisely at
the junction between the gene segment and the RSS (figure 6). DNA repair
complexes then repair the doubleanded breaks, but attach the two gene
segments to each other, and the two RSSs to each other. The region in
between the gene segments, which include the RSSs, ends up getting deleted in
the process. The DNA of the B cell is therefore irreversibly altered by
the recombination event.

According to Behe, an antibody-diversity system requires
three components: the antibody genes themselves, the recombination signal
sequences (RSS), and the recombination activating genes RAG-1 and
RAG-2.
"In the absense of the [RAG] machine, the parts never
get cut out and joined. In the absense of the signals, it's like
expecting a machine that's randomly cutting paper to make a paper doll.
And, of course in the absence of the message for the antibody itself, the
other components would be pointless." (Page 130).
Behe paints a pretty dire picture for how a process like
this could possibly have originated in Darwinian fashion. He goes even
further to state:
"The problem of diversity runs headlong into the
requirement for minimal function. A primitive system with only one or a
few antibody molecules would be like the propeller turning at one revolution
per day: not sufficient to make a difference... Because the likelihood
is so small for the shape of one antibody being complementary to the shape of
a threatening bacterium -- perhaps one in a hundred thousand or so -- any
animal that spent energy making five or ten antibody genes would be wasting
resources..." (Page 130-1).
The beneficial innate receptors
Behe is totally wrong to assert that receptors with
single specificities are useless to the immune system. There are
many non-rearranging, innate receptors very important to host
defense, such as the LPS receptor. Others include the glycan receptor, the
mannose receptor, and the macrophage scavenger receptor; which together
recognize many different bacterial constituents. The activation of an
adaptive immune response begins about three days after the start of
infection. Until those three days have passed, the only thing keeping the
pathogens at bay is the innate immune system. Phagocytic cells like
macrophages and neutrophils express a wide array of innate receptors that allow
them to detect and engulf many types of pathogens before they have a chance to
multiply and overwhelm the adaptive immune system. Invertebrates, which
lack an adaptive immune response, are entirely dependent on innate receptors for
survival. What is key to remember is that bacteria are very different from
their metazoan targets. There are numerous surface molecules essential to
bacteria that are absent in animal cells. These differences have
been exploited by the innate immune system hundreds of millions of years
prior to the emergence of the adaptive immune system. It is baffling why
Behe would state that innature receptors are "not sufficient to make a
difference", given the overwhelming abundance of contradictory evidence.
It is clear that Behe did not consult an immunologist prior to publishing this
book.
Two birds, one stone
Assuming that a non-rearranging antibody-like gene is
beneficial, two important components had to appear during the evolution of
antibody diversity: the signal sequences and the RAG genes. Their
origin is indeed a mystery. However, many clues can be discovered merely
by comparing the mechanism of recombination to other biochemical
processes. The process of recombination itself bears a great deal of
non-trivial similarity to another process which has nothing to do with
immunity. In fact, this process is an important tool in the armory of the
microbial invaders that are the targets of the immune system. It is a
process utilized by bacteria for antibiotic resistance, and by retroviruses to
invade the genome of their hosts. This process is transposition.
A transposable genetic element is a region of DNA capable
of "jumping" from one location on the genome to another. These regions are
around the size of a gene, as they usually encode the protein that catalyzes the
transposition reaction. Flanking these regions are sequences that
facilitate transposition, usually consisting of inverted repeats. While
the precise mechanism of transposition varies from system to system, it
typically involves the nicking or cleavage of DNA at sites on both the target
and donor, then swapping the two strands to integrate the transposon into the
target DNA. This process can be conservative or replicative, meaning that
it may or may not leave a copy of the transposon at its former location.
Transposons have been found in numerous organisms, including bacteria, viruses,
corn, worms, and fruit flies. Their presence in such a wide variety of
lifeforms suggests that either they are functionally important, extremely
efficient parasites, or both. Not surprisingly, a role for mobile
elements has been implicated in evolution (Hall, Collis et al. 1999).
V(D)J recombination shares a lot of similarity to
transposition (Lewis and Wu 1997; Lewis 1999; Schatz
1999). The RAG genes are closely linked on the mammalian genome,
being only 8 kb apart (Oettinger, Schatz et al. 1990).
Additionally, each gene is encoded by a single exon. This type of genomic
organization is rare for higher eukaryotes, but common for genes contained
within transposons. Additionally, the RSS sequences are similar to
the inverted repeat sequences used by many transposons (Dreyfus 1992). The
reaction itself has many features similar to transposition (Spanopoulou, Zaitseva et al. 1996).
For instance, the cleavage reaction mediated by the RAGs leads to the formation
of a DNA hairpin intermediate structure (Roth, Menetski et al. 1992). Transposases,
such as the Tn10 transposase in bacteria, can also generate similar hairpin
intermediates (Kennedy, Guhathakurta et al. 1998). This
feature is also shared by retroviruses during integration (Van Gent, Mizuuchi et al.
1996). However, the strongest piece of evidence favoring a transposase
origin for V(D)J recombination was the demonstration of transposase activity by
RAGs in vitro (Agrawal, Eastman et al. 1998; Hiom, Melek et
al. 1998). Two research groups independently discovered that
purified RAG proteins in vitro could not only excise a DNA region
flanked by RSSs, but also reinsert that region into another location
(transposition). The ability to transpose a region of DNA to a new genomic
location has no use in V(D)J recombination, and does not occur in
vivo. With that discovery, there can be little doubt that the
similarities between the two processes are more than
coincidental.
How can we use this information to generate a model for
the origin of V(D)J recombination? Behe states:
"As scientists we yearn to understand how this
magnificent mechanism came to be, but the complexity of the system dooms all
Darwinian explanations to frustruation." (Page
139).
It's a good thing not all scientists are as easily
discouraged as Behe by the complexity of this system. Let's see what
others have come up with. The transposon model is currently the most
feasible model to explain the origin of V(D)J recombination. The model
essentially states that the RAGs were originally contained within a transposon,
and that this transposon integrated into a gene for a primordial antigen
receptor (Thompson 1995). Later the RAGs
were moved to another location on the genome, where their expression could be
better regulated. The initial integration event, in one step, inserted
both the RAG genes and the RSS sequences, and generated a rearranging antigen
receptor.
A more detailed model could proceed as follows: A
transposon containing the RAG genes and flanked by RSSs integrates itself
into the gene for a primordial antigen-receptor gene, splitting it
into two gene segments (V and J). The locus itself
is transciptionally inactive in most cell types, and prevents
the expression of the RAG genes and removal of the integrated
transposon. However, in a lymphocyte-like cell, the locus becomes
transcriptionally active, and the RAG genes express themselves and remove the
transposon, reuniting the two gene segments. The imprecise joining process
generates a level of receptor diversity that favors the organism bearing this
transposon, and its descendants thrive with the increased immune
capabilities. A gene duplication creates a second locus, and the two
lineages become the heavy and light chains. A second integration event by
another transposon splits the heavy chain's V segment into V and D
segments. Further gene duplications create additional V, D, and J gene
segments, giving the receptors multiple rearrangement targets and increasing
their diversity of antigen recognition.
Conclusion for
system II
The similarity between RAGs and transposases is not a
recent discovery. Similarities between the two systems were known as early
as 1979, when the RSSs of the kappa light chain J segments were first sequenced
(Sakano,
Huppi et al. 1979). In the discussion section, the author stated, "We
propose that such a mechanism was initiated when an IS-like [insertion sequence]
DNA element was accidentally inserted into one of the multiple V DNA copies of
an ancestral polymeric gene,.." While the model has been greatly refined
since then, the basic concept remains the same. Why would Behe neglect to
mention the transposon-origin model? Sakano et al is cited by 706 other
research articles, clearly it is taken seriously by the scientific community[iii]. Instead, Behe cites a
two-page commentary in PNAS (Bartl, Baltimore et al. 1994)
as the "best" article discussing the molecular origins of V(D)J recombination,
to which he describes as making "a valiant stab at accounting for the
components, but in the end, it is a hop in the box with Calvin and
Hobbes." He also adds, "their proffered solutions are really just a
disguised shrug of their shoulders." He makes no attempt to address their
model, dismissing it with a wave of his hand. He also fails to appreciate
the context of the article, which is primarily a commentary on how the discovery
of TCR genes in sharks two months earlier had influenced current opinion.
They did not delve into the details of the transposon-origin model not only
because the scope of the article was so focused, but also because so many
articles before had described it. Their aim was to add to the discussion,
not reiterate it. They assumed the reader would already be familiar with
the basics of that model. Rather than stopping after reading one article,
perhaps Behe should have used the "related articles" tool on PubMed and read one
of the other 220 articles listed[iv]. For example, the article "New
insights into V(D)J recombination and its role in the evolution of the immune
system", is cited by 90 other articles (Thompson 1995). This
9-page review article provides a much more in depth description of the
transposon-origin model. Behe was quite incorrect when he stated:
"We can look high or we can look low, in books or in
journals, but the result is the same. The scientific literature has no
answers to the question of the origin of the immune system." (Page
138)
This proclamation is absurd, and academically
irresponsible. Behe singles out a commentary for scrutiny, ignoring the
wealth of literature on the topic, and concludes no answers exist. He
should have looked harder, or asked an immunologist. Hypotheses put forth
at the time of Darwin's Black Box's publication have
since been confirmed, and the transposon-origin model is now quite robust,
especially with the discovery of the transposase activity in RAGs. If only
ID could claim similar progress in the last 6 years.
IC system III: The complement cascade
The complement system is a cascade of serine proteases
initiated by the detection of foreign agents, and resulting in several different
effector responses. A serine protease cascade is system of proteins that
can sequentially activate each other through cleavage, and possess a serine
residue in their catalytic site. They function analogously to a chain
letter, where the first protease cleaves and activates several target proteases,
who in turn cleave several of their targets, and so forth. The result is
an exponential amplification of activity, set off by as few as a single
activated protease. In mammals, the cascade as a whole uses around 30
different proteins, which are all constituitively expressed in the blood
serum. In fact, complement proteins are believed to comprise a higher
percentage of blood plasma proteins than any other group of proteins.
There are essentially three ways to initiate the cascade,
called the classical, alternative, and lectin pathways (Figure 7, top
boxes). While the classical requires the binding of antibody to antigen,
the alternative and lectin pathways do not. The classical pathway was the
first of the three to be discovered, due to its ability to "complement" the
activity of antibody molecules in eliminating red blood cells in cross type
blood transfusions. When multiple antibody molecules recognize its target,
the complement factor C1 (which is a complex of three different proteins, C1q,
C1r, and C1s), binds to the antibody and becomes activated. It can then
catalyze the cleavage of C4 into C4a and C4b. C4b contains a highly
reactive thioester group, which causes it to covalently bind to proteins near
it, which presumably includes the antibody's bound target, like the surface of a
bacterium. C2 can then attach to C4b, and is then cleaved by C1 into C2a
and C2b. C2b and C4b then form the second serine protease in the cascade,
called C3 convertase. This complex induces the cleavage of another factor,
C3, into C3a and C3b. C3b can group with the C4b/C2b complex to form a C5
convertase complex, which cleaves C5 into C5a and C5b. C5b attracts the
complement proteins C6, C7, C8 and C9. This group of proteins, C5b through
C9, forms a membrane attack complex, which is basically a pore on the surface of
the bacterium. The formation of several of these pores disrupts the
osmotic balance of the bacterium, resulting in its lysis.

Antibodies are not required for complement activation
Aside from his typical complaint that this system is "too
complex to have evolved", Behe does little to actually specify what makes the
complement pathway irreducibly complex, and does not give a list of essential
components. He spends a lot of time describing the classical pathway, and
its requirement for antibody-binding. By focusing on the classical
pathway, Behe implies that antibody-binding is essential to complement
activation. This could not be further from the truth. Organisms
lacking an adaptive immune response, such as lampreys or tunicates, do posses
complement factors and have an efficient complement system (Nonaka and
Takahashi 1992; Smith, Azumi et al. 1999). These animals do
not use the classical pathway of activation, but instead rely on the
lectin and alternative pathways. In mammals, the lectin pathway is
initiated by the binding of a protein called MBP (mannose-binding protein) to a
mannose-containing saccharide on the surface of many types of gram-negative
bacteria (Matsushita, Endo et al. 1998). This
leads to the binding of two proteins, MASP-1 and MASP-2 (MBP associated serine
protease), which catalyze the cleavage of C4 and C2 into C4a/C4b and C2a/C2b,
then join the classical pathway at the C3 convertase. Lampreys and
tunicates have similar lectin pathways as mammals. Interestingly, the
complex of MBP/MASP-1/MASP-2 strongly resembles the C1q/C1r/C1s complex, in
sequence, structure, and function.
The alternative pathway is much more simple to activate,
utilizing the fact that an intermediate step in the classical and lectin
pathways, the cleavage of C3, can often occur spontaneously. As it turns
out, C3b can associate with a protein called Factor B (Bf) to form a C3
convertase, eliminating the need for C4b and C2b entirely (Xu, Narayana et al. 2001). Factor B binds to C3b, and is then cleaved into its active form, Bb, by
a serine protease called Factor D. The mechanism for activation is quite
similar to C4b and C2b, and C3b is very similar in structure to C4b, and Bb is
very similar to C2b. Additionally, Factor D has sequence similarity to
trypsin, a very common serine protease. Behe leaves out the lectin pathway
entirely, and only briefly mentions the alternative. Why? It is
clear that the origins of the complement system lie within one of these two
activation pathways, so their function and history should be focused upon.
By spending so much time with the classical pathway, Behe makes the system's
origin appear more complicated than it actually may be.
The multiple, independent functions of the complement
system
Behe defines an irreducibly complex system as a system
that contains multiple essential parts, where the elimination of any one of
these parts results in the loss of the function of that system. However,
in the case of the complement system, their function is somewhat unclear.
Behe never states specifically what the function of this pathway is, but implies
that it is to lead to the formation of the membrane attack complex (MAC),
thereby causing the lysis of the bacterium. However, the branch of the
cascade leading to MAC formation (called the lytic pathway) represents only one
particular effector function of complement. In general, the complement
pathway leads to three types of effector responses; inflammation, opsonization,
and MAC formation (Figure 7, bottom boxes). While the cleavage of C2, C4,
C3, and C5 is important for their function in propagating the cascade, the
cleavage products C4a, C3a, and C5a are all anaphylatoxins. These function
as local mediators of inflammation, which can recruit innate immune cells like
phagocytes to the site of infection. C2b, C4b, C3b, and C5b can also serve
as opsonins. That is, they can facilitate the phagocytosis of their bound
targets by binding to receptors on the surface of phagocytes. Since the
lytic pathway has so far not been detected in organisms lacking an adaptive
immune system, the opsonizing function of complement is presumably its original
function. However, Behe doesn't even mention the complement system's
function in opsonization. Nor does he recognize the fact that many
organisms that lack both the classical pathway of activation and the lytic
pathway still have a robust complement-mediated immune system. It would
seem that his intention here is to snowball the reader with endless descriptions
of complexity, rather than specifying the key features that directly relate to
evolution and origins. Additional discussion of the complement system as
an irreducibly complex system can be found in the talkorigins article, " Is the Complement
System Irreducibly Complex?"
Molecular and genetic
comparison of the innate immune systems of different organisms
"Comparing sequences might be a good way to study
relatedness, but the results can't tell us anything about the mechanism that
first produced the systems." (Darwin's Black
Box, Page 138).
Sequence comparison is one of the first steps in
analyzing the function of a gene. By comparing the sequences of a gene
between two distantly related groups, regions important for function can be
identified for future experiments. This is a commonly used strategy in any
lab. Comparing the sequences of a family of genes within multiple groups
of organisms can reveal a wealth of information on the evolution of that family,
including which members appeared through gene duplications and when. All
of this information can be used to infer the function of the ancestral state of
the system, and how it might have originated. This is a much more
effective means of examining the origin of a system than Behe's apparent method,
removing components and testing for loss of function. Removing components
is not like rewinding a tape. It does not necessarily tell us what the
ancestral state was like.
While a fish probably looks more like the common ancestor
of mammals and fish than we do, it is still over 100 million years more evolved
than its ancestor. However, we can still look at the features of a fish's
immune system and compare it to our own. If a similar feature is observed,
it most likely arose prior to the divergence of the two groups. By
comparing humans to other groups, we can begin to trace back our evolutionary
roots. The following section attempts to explore the innate immune systems
of other organisms in relation to our own. It begins with more distantly
related organisms and continues through to the jawed vertebrates. Though
separated by 450 million years of evolution, even cartilaginous fish like sharks
and manta rays have essentially the same complement pathway as humans (Nonaka and
Smith 2000). Refer to figure 8 to see the relationships between each
group.
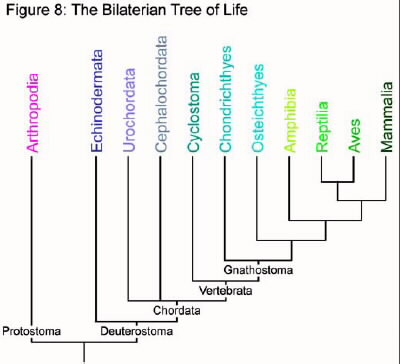
Arthropods
(phylum Arthropoda)[v]
Insect immunity is mediated primarily through the
secretion of antimicrobial peptides, whose exact functions are unknown.
While insects do not have a complement system, they do possess several different
types of lectins and serine proteases, which have features similar to complement
and blood-clotting. Some lectins are able to induce coagulation upon
recognition of certain microbial surface constituents (e.g. LPS) (Muta and Iwanaga
1996). Coagulation is a serine protease-dependent process that forms
an insoluble matrix surrounding infectious particles, which are then cleared
from the hemolymph (insect "blood"). This process has many features in
common with blood-clotting. Furthermore, lectins with fibrinogen-like
domains, such as Tachylectin 5A and 5B (TLs-5) are also involved in this process
(Gokudan, Muta et al. 1999). Fibrinogen is
one of the key players in vertebrate blood-clotting. TLs-5 may represent
an evolutionary link between immunity and blood-clotting (Kairies, Beisel et al.
2001).
α2-macroglobulin (α2M), a protease
inhibitor, can regulate the hemolytic activity of proteolytic enzymes in the
hemolymph (insect "blood"), such as the lectin limulin (Armstrong and Quigley
1999; Swarnakar, Asokan et al. 2000). It
contains a protease sensitive region, which serves as "bait" for a wide variety
of proteases. Once cleaved, a highly reactive thioester bond is exposed,
which covalently attaches α2M to the protease. α2M is then able to
form a "cage" around the protease, which is then cleared from the hemolymph
through receptor-mediated endocytosis (Melchior, Quigley et al.
1995). As such, α2M can not only regulate its own proteases, but also
deactivate pathogenic proteases secreted by infectious agents. α2-macroglobulin shows remarkable homology to C3, C4,
and C5, suggesting that α2M may represent an evolutionary precursor to C3/C4/C5
(Sottrup-Jensen, Stepanik et al.
1985). Remarkably, a thioester protein similar to α2-macroglobulin was
discovered recently in mosquitos, called aTEP-I (Levashina, Moita et al.
2000). aTEP-I has complement-like opsonization activity, and could
represent an evolutionary precursor to C3. Furthermore, it had weak
binding activity in the absence of proteolytic activation, suggesting that a
more ancestral form of this protein could function without being cleaved.
Other immune-related molecules include the pentraxin
lectin family. One of these components, C-reactive protein (CRP), is
present in vertebrates, and there can activate the classical complement pathway
(Armstrong, Armstrong et al. 1993).
Overall, it appears that the very beginnings of complement-mediated immunity as
well as blood-clotting may have begun before the proteostome/deuterostome
split. Echinoderms (phylum Echinodermata)[vi] Recently, a C3/C4/C5-like gene was discovered
in the purple sea urchin strongylocentrotus
purpuratus (Al-Sharif, Sunyer et al. 1998). It was
most similar to C3, and was therefore named SpC3. SpC3 is expressed
exclusively by phagocytic coelmocytes, and is upregulated upon exposure to LPS
(Clow, Gross
et al. 2000). SpC3 serves primarily as an opsonin, and inhibiting SpC3
inhibits the phagocytic ability of their immune system. Additionally, a
C2/Bf-like gene was also identified, called SpBf (Smith, Shih et al.
1998). SpBf is equally related to mouse C2 and mouse Bf, suggesting
that the two diverged after sea urchins split off from the other
deuterostomes. Because of the presence of these two genes, and their joint
function in opsonization, it is believed that sea urchins can activate
complement through the alternative pathway (Smith, Clow et al.
2001). No other complement genes have so far been found, yet despite
that absence, sea urchins have an effective complement-mediated immune
system. Presumably, the positive feedback loop of the alternative pathway
gave the ancient deuterostomes the ability to rapidly induce the phagocytosis of
pathogens, a key advantage over simpler immune systems.
Urochordates (phylum
chordata, subphylum urochordata)[vii] Like sea urchins, a C3 homologue has been
identified in the solitary ascidian halocynthia
roretzi, called AsC3 (Ji, Azumi et al. 1998). And like SpC3,
ascidian C3 plays a strong role in opsonization, indicating that ascidians
possess the alternative pathway (Nonaka, Azumi et al. 1999). In addition,
two MASP-like genes were also discovered, which can cleave and activate AsC3 (Ji, Azumi et al.
1997). Putative MBP and Bf genes have also been recently discovered
(Nair, Pearce
et al. 2000; Nonaka and Miyazawa 2002). AsBf's serine
protease domain has sequence similarity to MASP-3, suggesting a
relationship. Furthermore, a class of lectins called ficolins has been
shown to be able to activate the complement pathway in conjunction with MASPs,
suggesting that ascidians possess a lectin pathway (Matsushita, Endo et al.
2000). However, the ascidian lectin pathway activates C3 directly, as
they do not appear to possess C4 or C2.
Ficolins are proteins composed of basically two domains,
a fibrinogen-like domain used for carbohydrate binding, and a collagen-like
domain used to activate the MASPs (Matsushita and Fujita 2001). MBP also
possesses a collagen-like domain very similar to ficolins, but does not have a
fibrinogen-like domain. In addition to activating complement, ficolins and
MBP can act as opsonins through their collagen-like domains. This suggests
that ficolin or MBP may have been added to the already existing alternative
pathway to form the lectin pathway in an ancestor to the urochordates. Cephalochordates (phylum chordata, subphylum
cephalochordata)[viii]
Amphioxus (also called lancelets) of the subphylum
cephalochordata are considered to be the closest extant relative to the
vertebrates. Their basic shape and genomic organization resemble
vertebrates, but they are much simpler. As a result, they are often
studied to shed light on key features of vertebrate development. Although
immunological research on amphioxus is just beginning, a key discovery has
already been made. A C3-like gene and a C6-like gene were found in the
amphioxus, Branchiostoma belcheri (Suzuki, Satoh
et al. 2002). While the C3-like gene is not surprising, the presence
of a C6-like gene is. C6 is a component of the cytolytic membrane-attack
complex (MAC), which is involved in the lysis of foreign pathogens. This
strongly suggests the presence of a lytic pathway in cephalochordata. The
complement proteins C6, C7, C8, and C9 all share key structural motifs, which
may indicate a common origin. Phylogenetic comparisons of amphioxus C6 to
human C6, C7, C8, and C9 suggest that amphioxus C6 is not that common
ancestor. These results would therefore predict the existence of
additional MAC components in amphioxus, and that the lytic pathway has a more
ancient evolutionary origin Jawless fish (phylum
chordata, subphylum vertebrata, class agnatha, order cyclostomata)[ix]
The jawless fish, or agnathans, of the class cyclostoma
has only two extant groups, lampreys and hagfish. They represent the most
ancestral vertebrates. Despite extensive searching, no rearranging antigen
receptors or MHC genes have been found in cyclostomes. On the basis of
this negative evidence it is generally assumed that they do not possess an
adaptive immune system, making them the only group of vertebrates without
one. However, and a C3 homologue has been detected in lamprey, as well as
a Bf/C2 homologue, and a MASP homologue (Nonaka, Fujii et al. 1984;
Nonaka,
Takahashi et al. 1994; Endo, Takahashi et al. 1998). The lamprey
Bf/C2 gene is equally related to mouse Bf and mouse C2, indicating that the gene
duplication that produced mouse Bf and C2 occurred after the cyclostomes
diverged from the jawed vertebrates. Lamprey C3 is more similar to mouse
C3 than to mouse C4 and C5. However, a lamprey C4/C5 homologue has not yet
been identified, so it remains unclear whether the cyclostomes emerged before or
after the C3/C4/C5 gene duplications (Nonaka 2001). Interestingly, lamprey C3a
is very similar to mouse C3a, while ascidian and sea urchin C3a are not.
Since mouse C3a serves as an anaphylatoxin (an inflammatory mediator), it
suggests that this ability evolved in an ancestor to the cyclostomes.
Additionally, a C5a-like anaphylatoxin ability has been reported in hagfish (Newton,
Raftos et al. 1994). The lytic pathway of the complement system has
not yet been observed in cyclostomes; however, a gene was cloned recently in
hagfish that bears remarkable similarity to CD59 (dos Remedios, Ramsland et al.
1999). In mammals, CD59 is an inhibitor of the lytic pathway,
preventing lysis of self cells. The cloned gene has 33% amino acid
similarity to CD59, suggesting that it is indeed a homologue. Recent data
also suggests that lampreys have lymphocytes, but since lymphocytes in all other
vertebrates utilize rearranging antigen receptors, their role in lamprey
immunity remains unclear (Shintani, Terzic et al. 2000).
In summary, it appears that the jawless fish contain all
the similar immune related genes and pathways that sea urchins and urochordates
possess. However, several new features have appeared, such as the use of
anaphylatoxins, the possible appearance of lymphocytes, and preliminary evidence
that suggests the existence of the lytic pathway. Unfortunately the next
group of organisms, from the class Placodermi, are all extinct. Unless we
develop the technology to extract DNA from their remains, their immune systems
will most likely remain a mystery.
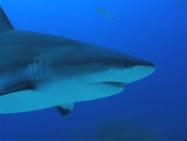
Cartilaginous fish (phylum
Chordata, class Chondrichthyes)[x]
The cartilaginous fish generally includes three groups of
organisms; sharks, skates, and rays. They are classified due to their
cartilaginous skeletons. They also possess jaws, and are the most
ancestral group of the jawed vertebrates, or gnathostomes. They are also
the most ancestral organisms that possess an adaptive immune system. Their
innate immune systems possess all three complement activation pathways
(classical, alternative, lectin), and their complement can serve as
anaphylatoxins, mediate opsonization, and activate the lytic pathway (Smith
1998). While not all components homologous to the mammalian complement
system have yet been identified, it is clear that their system is as complete as
ours. It is likely that in the coming years all components will be
discovered. In that sense, the story of the origins of complement-mediated
immunity ends with the cartilaginous fish.
Model for
the evolution of the complement system
Any model for the origin of the complement system must
begin with the evolution of an α2-macroglobulin-like
gene into a C3-like gene. C3 is the key molecule in the complement
system. It can interact with as many as 25 other proteins, and is the
point of junction for all three activation pathways (Sahu and Lambris 2001).
C3's strong sequence similarity to α2M, which is not part
of the complement system and presumably more ancient, clearly suggests a
possible origin. Additionally, C3 is not a serine protease, and an
explanation is required for how it became associated with serine
proteases. The fact that α2M is a protease
inhibitor and serves as a target for bacterial proteases also suggests how this
may have come about.
In the scientific literature, the models that are
proposed for the evolution of the complement system are not nearly as detailed
as the following model. The reason for that is simple. Behe is the
only person that is requiring that level of detail in order to be convinced that
it could have evolved. It would be similar to hypothesizing that a
particular football team would win the superbowl, but then a critic would demand
details like how many first downs the team would get, the total yardage
accumulated by both offenses, whether Gatorade would be poured upon the coach,
etc. That level of detail is not justified based on what information is
currently available. Therefore, the following model is not meant to be
completely accurate. It's an attempt to synthesize the current information
to provide an example of what could have happened,
not what did happen. According to Behe, a
Darwinian explanation for the evolution of an irreducibly complex system is a
logical impossibility. Therefore, to falsify his IC to ID inference, we
need not prove the model. We need only to provide a reasonably plausible
model.
It should also be mentioned that this model contains many
holes, many gaps that need to be filled by experiments or other evidence.
However, the model also makes predictions, some of which can lead to future
research. Future experiments may disprove this model. That is
perfectly fine, it will just allow us to generate a new model that will fit the
new evidence. This new model will make further, more specific predictions,
and the cycle will continue until all remaining holes have been filled.
We're at the beginning of the genomic era, and the comparison of sequences will
allow us to test and refine this model in ways that would have previously been
impossible.
The origin and evolution of the
complement system
The evolution of complement begins with an ancestor to
protease inhibitor α2-macroglobulin. It was constituitively
expressed in the hemolymph of the early bilaterians around one billion years
ago. The key to its function was the protease-sensitive "bait" region,
which when cleaved exposed a highly reactive thioester site, which would
covalently bind to whatever protease cleaved it. After attachement, the
α2M/protease complex would be cleared from the system
through phagocytosis. This would provide some protection for the host
from various types of bacterial proteases.
Numerous carbohydrate-binding lectins are present in
the hemolymph of all bilaterians. Some of these can recognize bacterial
cell wall constituents (e.g. LPS) and serve as opsonins. Shortly after
the deuterostomes split off from the protostomes, a serine protease domain was
attached to a carbohydrate-binding domain through exon shuffling. This
protein, a precursor to ficolins, could bind to bacteria, then cleave and
activate the α2M-like protein. Because α2M binds covalently
to its target, this would give a much stronger opsonizing activity than
through lectin binding alone. A gene duplication of α2M and subsequent
differentiation through random mutation followed by natural selection would
optimize the interaction between the ficolin precursor and the protein that
would eventually become C3. Because of the reactivity and lack of
specificity of the C3 precursor, various inhibitors of C3 began to evolve on
the surface of host cells, preventing them from being engulfed.
The key step in creating the complement pathway began
sometime before the echinoderms branched off from the prechordates. The
ancestral C3 protein evolved an affinity for a serine protease, which would
attach to C3b after cleavage. By localizing a serine protease near the
site of C3b attachment, the host could generate more C3b in close proximity to
the pathogen. With the addition of the ancestral Factor B, the positive
feedback loop that would be the hallmark of the complement pathway was
born. This loop gave the ancestral complement system the ability to
deposit large amounts of C3b onto the surface of pathogenic molecules very
rapidly. Additional evolution produced greater specificity through an
increased affinity for C3b by itself, and better regulation by the requirement
for C3b-binding for Factor B activation.
Because of the numerous regulatory molecules
preventing complement activation against self-molecules, the requirement for
lectin activation of complement became increasingly less stringent. C3
could evolve to become more and more spontaneously reactive, as low levels of
serum C3b were tolerated. With the addition of a dedicated activator in
Factor D, the alternative pathway was created.
After the echinoderms branched off from the chordates,
more sophisticated lectin complexes evolved. The MASPs were created,
possibly from a gene duplication of Bf (Nonaka and Miyazawa
2002). They originally interacted with ficolins, but through an exon
shuffling event joining a ficolin's collagen-like domain to a CRD,
mannose-binding protein (MBP) was created. Sometime before the emergence
of the jawed vertebrates, a genome-wide duplication event created additional
copies of C3 and Bf. These would later evolve into C4 and C2. The
lectin pathway would then utilize these new genes, increasing their affinity
for C4 and C2, and decreasing their affinity for C3 and Bf. By adding an
additional step, the amplification power of the protease cascade was
increased.
When the adaptive immune system appeared with the
jawed vertebrates, the complement system was quick to take advantage of
it. Gene duplications of MBP and the MASPs created C1q, C1r, and
C1s. Once C1q evolved the ability to bind to immunoglobulins instead of
carbohydrates, the joining of these two pathways was complete. The
combined system possessed the specificity of immunoglobulins, and the effector
response of complement.
The addition of the lytic pathway to the complement
system could have occurred before or after the creation of C4. One of
the major features of any model of complement evolution is the notion that C4
and C5 were created from gene duplications of C3. However, it remains
unclear whether C4 was created first, or C5. In jawless vertebrates,
only C3 is present. In jawed vertebrates, both are present.
Regardless, at some point either before or after the emergence of the jawed
vertebrates, the lytic pathway was added to the complement system. C5
was initially created, and had opsonizing activity (C5b), and anaphylatoxin
activity (C5a). A molecule with a perforin domain, which is the key
domain in mediating cell lysis, appeared. In time, this perforin-like
gene evolved into C6, C7, and C8, and could lyze enucleated cells. C9
was added as a final step to form a pore capable of lysing nucleated cells.
What predictions can be made from this model? One
interesting feature of this model is that it suggests that the ancestral
complement system required a lectin pathway-like activation mechanism. If
true, then we would predict that complexes with lectin and serine protease
activities would be found in the complement system of echinoderms.
Currently, only C3-like and Bf-like genes have been found. It is possible
that the early echinoderms had a lectin pathway then lost it, but this is
unlikely. The ancient lectin pathway need not contain proteins as complex
as the MBP/MASP complex. Those may have appeared later, before the
urochordates and vertebrates split. In the coming years, if no putative
lectin activator is found, then that could be considered evidence against this
model. If that happened, then any model that does not require lectins as
the initial activator will be supported.
While much of this model is speculative, it is based on
the comparison of the functions and genetic sequences of complement proteins in
different organisms. There are still many gaps to be filled in, but the
foundation of the evolutionary pathway is clear. Much of the complement
system was formed through a series of gene duplication events, co-opting the
existing genes to add additional layers to this proteolytic cascade. This
is not an uncommon idea in evolutionary biology, and there is much evidence to
suggest that many serine protease cascades were generated through similar means
(Krem and Di
Cera 2002). While one could demand more evidence to support this
story, it's a much better story than what we could have come up with five years
ago, which was much better than ten years ago, and so on. There is a trend
of increasing information and knowledge that makes the story more precise, with
fewer holes. To seek an answer to a particular gap in our knowledge is
what science is all about. To doubt that the gap can be filled is
unjustified. To claim that the existence of a gap is evidence that it
cannot be filled is absurd.
Conclusion
The mammalian immune system is indeed a complex
system. It may very well be irreducibly complex, as Behe suggests.
However, complexity by itself does not eliminate evolution. By now it
should be clear to the reader that in each system discussed in Chapter 6 of Darwin's Black Box, there are models for their origins
and ample evidence to support those models. He fails to acknowledge the
strength of evidence supporting evolutionary models, or dismisses them without
justification. Behe makes critical errors that distort his
analysis. Let's review the systems described by Behe and their
supposed "evolvability":
Clonal Selection - Behe
lists three components as part of the clonal selection system's "core":
the membrane-bound immunoglobulin, the signal transduction machinery
(messenger), and the secreted Ig. According to him, none of these
components can serve any function without the others. Behe is
incorrect. The components of the signal transduction pathway exist in
animals, such as insects, that do not possess the clonal selection
system. Numerous innate receptors exist that induce a signal, but do not
switch from a membrane-bound form to a secreted form upon activation.
There are receptors that are expressed in membrane-bound form and secreted
form, but are not induced to do so. Lastly, there exists a receptor with
extreme homology to immunoglobulins and uses very similar signaling proteins,
but does not switch forms. We can observe in nature functional systems
that lack one or more of Behe's "core" components. Whether or not those
components are essential for function in the clonal selection system has
nothing to do with how that system arose.
V(D)J Recombination - The
"core" of the V(D)J recombination system consists of the antigen receptor
genes, the recombination machinery, and the recombination signal sequences
(RSSs). While a non-rearranging antigen receptor with clear homology to
the immunoglobulins has yet to be discovered, there exist many innate
receptors that can protect the host from invasion. When Behe states that
"A primitive system with only one or a few antibody molecules would be .... not
sufficient to make a difference", he is dead wrong. Insects,
echinoderms, tunicates, lancelets, and agnathans have all been able to survive
without the benefit of an adaptive immune system, relying on an array of
innate receptors specific to common bacterial surface constituents. As
for the recombination machinery, and the RSSs, Behe is correct to state that
one is useless without the other. However, the proteins with homology to
the RAGs, and sequences quite similar to the RSSs can be observed operating in
bacterial and viral transposons. The current model that the adaptive
immune system originated when a transposon inserted itself into an innate
antigen receptor is alive and well. Since the publication of Darwin's Black Box in 1996, this model has all but
been confirmed by the discovery of transposase activity in the RAG proteins.
The complement system -
Behe's largest error in chapter 6 is choosing the complement system as an
example of how evolution cannot produce irreducible complexity. He not
only neglects to define which components form the "core", but lists nearly
every protein involved in the pathway, suggesting that all are required for
minimal function. He acknowledges only one of several functions of the
complement system. He even implies that the system is dependent on
antibodies. The discovery of subsets or precursors of the complement
system in invertebrates lacking antibodies dispels that notion. The fact
that these invertebrates' complement systems do not have the one function he
defined for it shows how faulty his IC logic is. However, whether or not
the system is irreducibly complex is irrelevant. The evidence for its
evolution is undeniable. There is a trend of increasing numbers of
components of the complement system going up the tree of life.
Arthropods have none, Echinoderms have a pair, tunicates have more, and so
forth. At what point along this evolutionary route does Behe think that
the barrier to evolution lays?
The errors made by Behe in Chapter 6 are not mere
oversights. He has taken faulty assumptions, and made very strong
conclusions based on them. Throughout the chapter Behe insinuates that no
research is being conducted on the evolution of the immune system, and that no
progress has been made. However, he is sorely mistaken. The
literature cited in this review represents only a tiny subset of the total
number of articles on the subject, and the total amount of research being
conducted. Why then, does he state, "We can look high or we can look
low, in books or in journals, but the result is the same. The scientific
literature has no answers to the question of the origin of the immune
system"? Although this statement is totally ludicrous, it would be unfair
to suggest that Behe has intentionally misled readers into thinking that the
scientific community is baffled by the immune system's complexity. It
would be fairer to say that he is merely ignorant of the abundance of research
and articles that exist on the subject, or that he does not understand
them. Behe is not an immunologist, and in the modern era of biology, it is
nearly impossible to be an expert in all fields. Furthermore, Darwin's Black Box is not a peer-reviewed article, but a
book meant for the lay reader. Should he be expected to know every detail
in the literature on immune system evolution? Absolutely. If Behe
wants the reader to believe that he is an authority on the subject, then yes, he
needs to know what every immunologist knows about the field. Is that
unfair? Perhaps the inaccuracy is due to the difficulties in conveying a
complex subject like the immune system to the lay reader. However, no
immunologist would say that Behe's analysis is accurate. The errors he
makes display a lack of familiarity with the field. He should not have
written a chapter on immune system evolution, nor made such strong
conclusions. Either that or he should have sent the manuscript to an
immunologist before publishing.
Irreducible complexity
versus comparative immunology as an analytical tool
Since we cannot look at the genes of our ancestors, we
must turn to our relatives. Armed with an understanding of how evolution
works, we can probe through the genetic sequences of different organisms, and
draw conclusions based on what is similar, and what is different.
Similarities suggest homology, and by generating phylogenies of genes and
families of genes from a large grouping of organisms, we can track when and how
quickly genes diverged. We can piece together what steps occurred in the
evolution of a system. We can use this information to generate models from
which to make hypotheses, then test them in the lab. Based on those
results, we can eliminate inaccurate models and fine-tune surviving ones.
Ultimately, we'll inch closer and closer to a more complete understanding of how
the system works, and how it arose. For example, the model proposed for
the origin of the complement system is based solely upon comparisons of
different organisms. In time, more information on the origins of the other
two systems will be revealed through comparative immunology, such as whether the
immunoglobulin evolved from the T cell receptor or vice versa. It is
startling that Behe would dismiss the use of comparative immunology, stating
that it "does not address in molecular detail the question of how immune systems
originated." Perhaps he should purchase a subscription to the journal Developmental and Comparative Immunology?
Behe is a biochemist, and prefers functional analysis
over the comparison of gene sequences. The approach he proposes to analyze
the "evolvability" of systems is a genetic one: Remove a component and see
what happens. He feels that this method, through which irreducible
complexity is identified, can reveal potential difficulties in the evolution of
a system that scientists have previously overlooked. In doing so,
irreducible complexity can potentially challenge the scientific community to
think of new solutions in molecular evolution. But is his method
useful? Does it actually reveal potential roadblocks? In each of the
three systems described by Behe in chapter 6, I have neglected to discuss
whether the systems are IC. Why? Because it doesn't affect how the
system could have evolved. Whether or not a particular component is
required for function in the current system does not necessarily mean it was
required in an ancestral system. C4 and C2 are required for
complement activation in mammals, yet invertebrates do not possess them.
The ability to switch from a membrane-bound form to a secreted form is essential
for immunoglobulins, but not T cell receptors (TCR). As has been
previously mentioned, Behe's argument is essentially an argument from
ignorance. He seems to require detailed knowledge of a system's origin
before he will consider the possibility that it evolved through "Darwinian"
evolution. If the absence of C4 and C2 had never been discovered in
invertebrates, would we conclude that the complement system could not have
evolved? If TCRs were not present in jawed vertebrates, would we conclude
that an antigen-receptor that was only membrane-bound could not serve a useful
function? Behe's method takes an "unevolvable until proven evolved"
approach, which will invariably lead to false positives. Interestingly,
the method used to disprove his inferences, comparative immunology, is dismissed
by Behe. Perhaps the reason he dismisses it so quickly is because it can
only serve to disprove him? The approach he proposes may be useful in
determining the function of a system, and how each component contributes to it,
but it is clearly useless in regards to origins.
Peer-review
The issue of peer-review, or lack thereof, is one that
often arises in discussions of the ID movement. In fact, no critique of an
ID article would be complete without mentioning it. No research article on
ID has ever been published in a reputable peer-reviewed journal. ID
advocates might suggest that the scientific community is biased against them,
and rejects their articles for political reasons. Whatever the reason, ID
advocates have instead opted for publishing popular books, which do not require
the approval of the scientific community. Should we take these books
seriously, since IDists would claim that they are being actively prevented from
publishing in peer-reviewed journals? Individuals familiar with biology
can judge the work for themselves, but lay readers cannot tell when they are
being told good science, and when they are being misled. They cannot judge
the accuracy of the science for themselves, at least not without great
effort. Ultimately, there is a degree of trust that must be earned between
author and reader. Trust that the information conveyed is accurate.
Before a scientific journal will accept an article for publication, they send
copies of the article to two or three scientists who are considered to be
experts in the particular field encompassed by the article. They review
the article with a fine-toothed comb, and point out any weaknesses and errors
the authors may have made, and suggest experiments to strengthen it. The
editor of the journal will decide whether or not to consider the article, and
tell the author what improvements need to be made before they will accept
it. Changes can be as subtle as changing a verb from "indicates" to
"suggests". Only after the improvements have been made will the article be
published. This system of peer-review ensures the high quality of research
presented by articles in the journal, and prevents gross errors from being
published. Darwin's Black Box does not present
any original research, but is a review of the existing literature with a focus
on origins. However, even though the research and knowledge presented has
been published by others in peer-reviewed journals, the accuracy of Behe's
analysis is so poor that it clearly would not have passed peer-review had it
been submitted to a journal. That is why peer-review is so important to
scientific progress, and why the issue is brought up time after time by ID
critics. Peer-reviewed journals stake their credibility on the
articles they publish. They have earned the respect and trust of their
readership.
Skepticism and motivation
Oftentimes in the evolution/creation debate, the question
of motivation arises. Proponents of evolution will state that the ID
advocates are religiously motivated, and the ID advocates will in turn state
that the evolution proponents are materialists, or dogmatic Darwinists.
This form of ad hominem argues against the
motivations of the individual, and distracts from the heart of their
arguments. This is generally considered a fallacy, and should be
avoided. However, much of the evidence of evolution is subject to
interpretation, and often requires the experience of an expert to
understand. If the lay person is unable to interpret the data for
themselves, they must rely on the opinion of the expert. In that case, it
may be important to understand the motivations and biases of those who claim to
be experts on the subject. In chapter 6, Behe writes as though he
considers himself an authority on immunology. However, many of his
statements display a lack of understanding of some fundamental concepts of
immunology. For example, he states that a non-rearranging antigen receptor
would be useless to the organism. As has been previously mentioned, this
is in stark contrast to what is observed. Behe also questions the validity
of certain molecular mechanisms for mediating evolutionary change, such as gene
duplication. He has demanded in each chapter detailed molecular
explanations for how these systems could have originated. Usually, when an
individual shows extreme skepticism for one concept, it is because they are
already convinced by an alternative concept. In Behe's case, that
would be Intelligent Design. However, his degree of skepticism toward
"Darwinian" evolution is not equally directed towards his own thesis. He
has offered no detailed mechanisms for how these systems could have been
designed, how they were implemented, and how often they occurred. In the 6
years following Darwin's Black Box's publication, he
has made no attempt to answer any of these questions, nor conducted any research
to further ID as a scientific concept. On the other hand, much progress
has been made towards increasing our understanding of how these systems arose
through evolution. In fact, about two-thirds of the articles cited here
were published after 1996. So why does Behe continue to espouse ID?
Why does he display such critical skepticism for evolution, when ID has nothing
to offer? Why does he make such strong conclusions based on erroneous
assumptions and an argument from ignorance? It is here that the issue of
motivation arises, not as a justification for dismissing his arguments, but as
an explanation for why he would continue to deny the abundance of evidence that
supports an origin of these systems through "Darwinian" evolution.
Issues that need to be addressed
If Dr. Behe reads this article, it would probably be
unwise for him to respond personally, as it would serve him no useful purpose
and just expose him to even more criticism. However, there may be others
who agree with his arguments and would like to respond. To those persons I
would ask that their response focus on the arguments specifically relating to
immunology, such as those presented in the body of the article, and not the more
generic arguments contained within the introduction and conclusion.
Arguing from incredulity should be avoided, especially in the absence of a
competing hypothesis. If the responder feels that a competing hypothesis
exists, please present it in as much detail as possible, or refer to an article
or book that contains the hypothesis. I would also request that any
attempt to respond to this article address the following concerns:
The definition of irreducible complexity is too vague
to be useful. Terms like "system", "function", "parts", "well-matched",
and "interacting" need to be clearly defined.
The T cell receptor is a rearranging antigen receptor
that lacks the ability to switch forms. Could the immunoglobulins have
evolved from T cell receptors?
Innate, non-rearranging receptors are beneficial to
the host. Behe is wrong to assert that "a primitive system with one or a
few antibody molecules would be...not sufficient to make a difference).
The similarities between the V(D)J recombination
machinery and transposases are not trivial. What explanation does Behe
have for why the RAG proteins are able to catalyze transposition reactions?
Behe did not list the essential parts for the
complement system. Additionally, he does not state what the function of
the complement system is. What are the essential parts? What is
the function?
How does the discovery of complement proteins in
invertebrates affect Behe's IC inference? Are antibodies required for
complement activation? If not, then are the components specific to the
classical pathway essential, as is suggested in chapter 6?
Behe often demands "quantitative calculations" for
evolutionary models. What quantitative calculations can be offered in
favor of ID?
What positive predictions can be made based on
irreducible complexity?
What research has been conducted on ID since Darwin's Black Box's publication? What research
has even been proposed?
Michael Behe presents a very bleak picture of how
evolution could have produced the systems described in chapter 6 of Darwin's Black Box. He makes several erroneous
assumptions in his analysis, some of which demonstrate a lack of understanding
of immunology. He only briefly mentions the current models, then dismisses
them without justification. He demands detailed explanations, but does not
offer any detailed alternatives in return. He scrutinizes evolutionary
theory, then bases his IC to ID inference on negative evidence and arguments
from ignorance. He makes no predictions, no hypotheses, and proposes no
research. Darwin's Black Box, and the concept
of irreducible complexity is essentially just a giant argument from ignorance
and a scientific dead end. Behe needs to be reminded of the old adage: "Absence of evidence is not evidence of absence".
Acknowledgements
Thanks to Andrea Bottaro, Nick Matzke, Russell Durbin, Mike Hopkins and the Talkdesign
crew.
References
Agrawal, A.,
Q. M. Eastman, et al. (1998). "Transposition mediated by RAG1 and RAG2 and its
implications for the evolution of the immune system." Nature (London) 394(6695):
744-751.
Al-Sharif,
W. Z., J. O. Sunyer, et al. (1998). "Sea Urchin Coelomocytes Specifically
Express a Homologue of the Complement Component C3." J
Immunol 160(6): 2983-2997.
Armstrong,
P. B., M. T. Armstrong, et al. (1993). "Involvement of alpha-macroglobulin and
C-reactive protein in a complement-like hemolytic system in the arthropod,
Limulus polyphemus." Molecular Immunology 30(10): 929-934.
Armstrong,
P. B. and J. P. Quigley (1999). "alpha2-Macroglobulin: An evolutionarily
conserved arm of the innate immune system." Developmental
& Comparative Immunology 23(4-5):
375-390.
Bartl, S., D.
Baltimore, et al. (1994). "Molecular Evolution of the Vertebrate Immune System."
PNAS 91(23):
10769-10770.
Behe, M. J.
(1996). Darwin's black box : the biochemical challenge to
evolution. New York, Free Press.
Clow, L. A., P.
S. Gross, et al. (2000). "Expression of SpC3, the sea urchin complement
component, in response to lipopolysaccharide." Immunogenetics 51(12):
1021-1033.
Daffre, S. and
I. Faye (1997). "Lipopolysaccharide interaction with hemolin, an insect member
of the Ig-superfamily." FEBS Letters 408(2): 127-130.
dos Remedios, N.
J., P. A. Ramsland, et al. (1999). "Identification of a homologue of CD59 in a
cyclostome: Implications for the evolutionary development of the complement
system." Developmental and Comparative Immunology 23(1): 1-14.
Dreyfus, D.
H. (1992). "Evidence suggesting an evolutionary relationship between
transposable elements and immune system recombination sequences." Molecular Immunology 29(6):
807-810.
Endo, Y., M.
Takahashi, et al. (1998). "Two lineages of MASP/C1r/C1s family in vertebrate."
Molecular Immunology 35(6-7): 349.
Fenton, M. J.
and D. T. Golenbock (1998). "LPS-binding proteins and receptors." Journal of Leukocyte Biology 64(1): 25-32.
Gokudan, S.,
T. Muta, et al. (1999). "Horseshoe crab acetyl group-recognizing lectins
involved in innate immunity are structurally related to fibrinogen." PNAS 96(18): 10086-10091.
Hall, R. M., C.
M. Collis, et al. (1999). Mobile gene cassettes and integrons in evolution.
Hiom, K., M.
Melek, et al. (1998). "DNA transposition by the RAG1 and RAG2 proteins: A
possible source of oncogenic translocations." Cell 94(4): 463-470.
Ishikawa,
G., K. Azumi, et al. (2000). "Involvement of tyrosine kinase and
phosphatidylinositol 3-kinase in phagocytosis by ascidian hemocytes." Comparative Biochemistry and Physiology Part A Molecular
& Integrative Physiology 125A(3):
351-357.
Janeway, C.
(2001). Immunobiology 5 : the immune system in health and disease. New York,
Garland Pub.
Ji, X., K. Azumi,
et al. (1998). "Opsonic complement C3 in the solitary ascidian, Halocynthia
roretzi." Molecular Immunology 35(6-7): 363.
Ji, X., K. Azumi,
et al. (1997). "Ancient origin of the complement lectin pathway revealed by
molecular cloning of mannan binding protein-associated serine protease from a
urochordate, the Japanese ascidian, Halocynthia roretzi." PNAS 94(12): 6340-6345.
Kairies, N.,
H.-G. Beisel, et al. (2001). "The 2.0-A crystal structure of tachylectin 5A
provides evidence for the common origin of the innate immunity and the blood
coagulation systems." PNAS 98(24): 13519-13524.
Kennedy, A.
K., A. Guhathakurta, et al. (1998). "Tn10 transposition via a DNA hairpin
intermediate." Cell 95(1):
125-134.
Krem, M. M. and
E. Di Cera (2002). "Evolution of enzyme cascades from embryonic development to
blood coagulation." Trends in Biochemical Sciences 27(2): 67-74.
Levashina,
E. A., L. F. Moita, et al. (2000). "Conserved role of a complement-like protein
in phagocytosis revealed by dsRNA knockout in cultured cells of the mosquito,
Anopheles gambiae." Cell 104(5): 709-718.
Lewis, S. M.
(1999). Evolution of immunoglobulin and T-cell receptor gene assembly.
Lewis, S. M.
and G. E. Wu (1997). "The origins of V(D)J recombination." Cell 88(2): 159-162.
Lindstrom-Dinnetz, I., S.-C. Sun, et al. (1995).
"Structure and expression of Hemolin, an insect member of the immunoglobulin
gene superfamily." European Journal of Biochemistry
230(3): 920-925.
Matsushita, M., Y. Endo, et al. (2000). "Ficolins
activate the lectin complement pathway." Immunopharmacology 49(1-2):
79.
Matsushita, M., Y. Endo, et al. (1998).
"Complement-related serine proteases in tunicates and vertebrates." Current Opinion in Immunology 10(1): 29-35.
Matsushita, M. and T. Fujita (2001). "Ficolins and the
lectin complement pathway." Immunological Reviews 180: 78-85.
Medzhitov,
R. and C. A. Janeway (1997). "Innate immunity: The virtues of a nonclonal system
of recognition." Cell 91(3): 295-298.
Melchior,
R., J. P. Quigley, et al. (1995). "Alpha-2-Macroglobulin-mediated Clearance of
Proteases from the Plasma of the American Horseshoe Crab, Limulus polyphemus."
Journal of Biological Chemistry 270(22): 13496-13502.
Muta, T. and S.
Iwanaga (1996). "The role of hemolymph coagulation in innate immunity." Current Opinion in Immunology 8(1): 41-47.
Nair, S. V., S.
Pearce, et al. (2000). "A collectin-like protein from tunicates." Comparative Biochemistry and Physiology B 125B(2): 279-289.
Newton, R. A.,
D. A. Raftos, et al. (1994). "Chemotactic responses of hagfish (Vertebrata,
Agnatha) leucocytes." Developmental and Comparative
Immunology 18(4): 295-303.
Nonaka, M.
(2001). "Evolution of the complement system." Current
Opinion in Immunology 13(1): 69-73.
Nonaka, M., K.
Azumi, et al. (1999). "Opsonic Complement Component C3 in the Solitary Ascidian,
Halocynthia roretzi." J Immunol 162(1): 387-391.
Nonaka, M., T.
Fujii, et al. (1984). "Purification of a lamprey [Lampetra japonica] complement
protein homologous to complement C3 of the mammalian complement system." Journal of Immunology 133(6):
3242-3249.
Nonaka, M. and
S. Miyazawa (2002). "Evolution of the initiating enzymes of the complement
system." Genome Biol 3(1):
REVIEWS1001.
Nonaka, M. and
S. L. Smith (2000). "Complement system of bony and cartilaginous fish." Fish & Shellfish Immunology 10(3): 215-228.
Nonaka, M. and
M. Takahashi (1992). "Complete complementary DNA sequence of the third component
of complement of lamprey: Implications for the evolution of thioester containing
proteins." Journal of Immunology 148(10): 3290-3295.
Nonaka, M., M.
Takahashi, et al. (1994). "Molecular cloning of a lamprey homologue of the
mammalian MHC class III gene, complement factor B." Journal of Immunology 152(5):
2263-2269.
Oettinger,
M. A., D. G. Schatz, et al. (1990). "RAG-1 and RAG-2, adjacent genes that
synergistically activate V(D)J recombination." Science
(Washington D C) 248(4962): 1517-1523.
Roth, D. B., J.
P. Menetski, et al. (1992). "Vdj Recombination Broken Dna Molecules with
Covalently Sealed Hairpin Coding Ends in Scid Mouse Thymocytes." Cell 70(6): 983-991.
Sahu, A. and J.
D. Lambris (2001). "Structure and biology of complement protein C3, a connecting
link between innate and acquired immunity." Immunological
Reviews 180: 35-48.
Sakano, H., K.
Huppi, et al. (1979). "Sequences at the somatic recombination sites of
immunoglobulin light-chain genes." Nature 280(5720): 288-94.
Schatz, D. G.
(1999). "Transposition mediated by RAG1 and RAG2 and the evolution of the
adaptive immune system." Immunologic Research 19(2-3): 169-182.
Shintani,
S., J. Terzic, et al. (2000). "Do lampreys have lymphocytes? The SPI evidence."
Developmental & Comparative Immunology 24(Supplement 1): S104.
Smith, L. C.,
K. Azumi, et al. (1999). "Complement systems in invertebrates. The ancient
alternative and lectin pathways." Immunopharmacology
42(1-3): 107-120.
Smith, L. C.,
L. A. Clow, et al. (2001). "The ancestral complement system in sea urchins." Immunol Rev 180: 16-34.
Smith, L. C.,
C.-S. Shih, et al. (1998). "Coelomocytes Express SpBf, a Homologue of Factor B,
the Second Component in the Sea Urchin Complement System." J Immunol 161(12):
6784-6793.
Smith, S. L.
(1998). "Shark complement: An assessment." Immunological
Reviews 166(0): 67-78.
Sottrup-Jensen, L., T. M. Stepanik, et al. (1985).
"Common evolutionary origin of .alpha.2-macroglobulin and complement components
C3 and C4." Proceedings of the National Academy of
Sciences of the United States of America 82(1):
9-13.
Spanopoulou, E., F. Zaitseva, et al. (1996). "The
homeodomain region of Rag-1 reveals the parallel mechanisms of bacterial and
V(D)J recombination." Cell 87(2): 263-276.
Su, X.-D., L. N.
Gastinel, et al. (1998). "Crystal structure of hemolin: A horseshore shape with
implications for homophilic adhesion." Science
(Washington D C) 281(5379): 991-995.
Sun, S. C., I.
Lindstrom, et al. (1990). "Hemolin: An insect-immune protein belonging to the
immunoglobulin superfamily." Science (Washington D C)
250(4988): 1729-1732.
Suzuki, M. M.,
N. Satoh, et al. (2002). "C6-like and C3-like molecules from the
cephalochordate, amphioxus, suggest a cytolytic complement system in
invertebrates." Journal of Molecular Evolution 54(5): 671-679.
Swarnakar,
S., R. Asokan, et al. (2000). "Binding of alpha2-macroglobulin and limulin:
Regulation of the plasma haemolytic system of the American horseshoe crab,
Limulus." Biochemical Journal 347(3): 679-685.
Takahashi,
H., G. Ishikawa, et al. (1997). "Cloning and tyrosine phosphorylation of a novel
invertebrate immunocyte protein containing immunoreceptor tyrosine-based
activation motifs." Journal of Biological Chemistry
272(51): 32006-32010.
Thompson, C.
B. (1995). "New insights into V(D)J recombination and its role in the evolution
of the immune system." Immunity 3(5): 531-9.
Thornhill,
R. H. and D. W. Ussery (2000). "A classification of possible routes of Darwinian
evolution." Journal of Theoretical Biology. 203(2): 111-116.
Van Gent, D. C.,
K. Mizuuchi, et al. (1996). "Similarities between initiation of V(D)J
recombination and retroviral integration." Science
(Washington D C) 271(5255): 1592-1594.
Xu, Y., S. V. L.
Narayana, et al. (2001). "Structural biology of the alternative pathway
convertase." Immunological Reviews 180: 123-135.
Endnotes
[i] One paper, "A classification of possible
routes of Darwinian evolution", by Thornhill and Ussery, defines Darwinian
evolution as, "Descent of organisms in which the following criteria are met: (i)
intergenerational differences are very much smaller than inter-specific ones;
(ii) no intervention by conscious agent(s) occurs; (iii) the frequency of
mutations or other heritable modifications is unrelated to functional utility;
and (iv) selection is the sole means by which heritable modifications are
accumulated to form functional structures" (Thornhill and Ussery
2000)
[ii] The Thornhill and Ussery paper does include a
discussion of irreducible complexity. However, because the term is not
mentioned in the title or abstract, it fails to appear on a PubMed search.
[iii] According to citation index at http://webofscience.com/
[iv] The PubMed citation index features a tool
which lists articles closely related to a selected article. Web readers
can click here
to see the list, or see http://www.ncbi.nlm.nih.gov/entrez/query.fcgi?db=PubMed
for details.
[v] For additional information on arthropods, see:
http://tolweb.org/tree?group=Arthropoda&contgroup=Animals
[vi] For additional information on echinoderms,
see: http://tolweb.org/tree?group=Echinodermata&contgroup=Animals
[vii] For additional information on urochordates,
see: http://www.personal.psu.edu/users/m/x/mxg276/bio110h.html
[viii] For additional information on
cephalochordates, see: http://www.personal.psu.edu/users/m/x/mxg276/bio110h.html
[ix] For additional information on cyclostomes,
see: http://tolweb.org/tree?group=Hyperoartia&contgroup=Vertebrata
[x] For additional information
on cartilaginous fish, see: http://www.ucmp.berkeley.edu/vertebrates/basalfish/chondrintro.html
This article was originally published at Talkdesign.org
|
 |